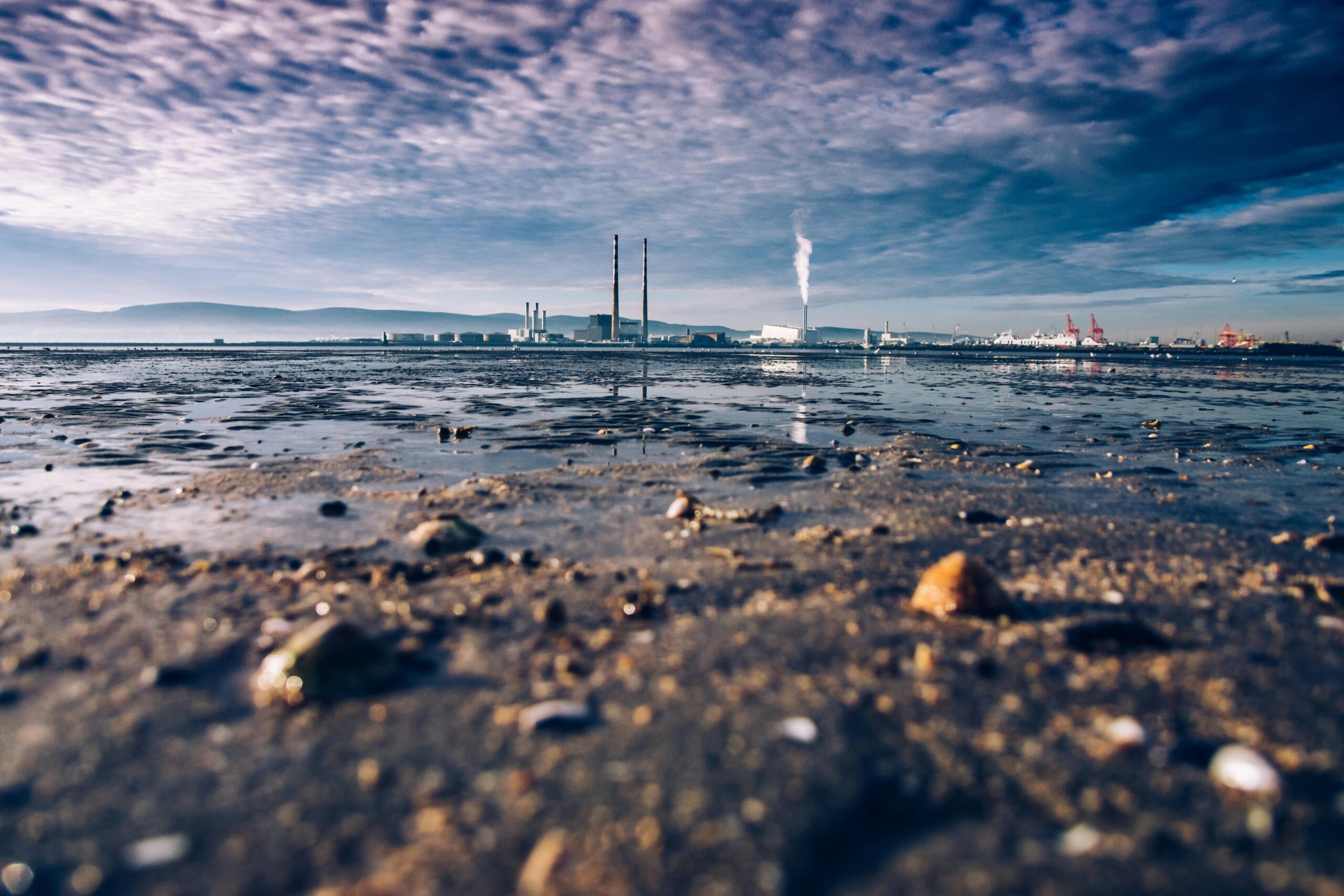
The Unseen Enemy: Impacts of Forever Chemicals on the Environmental and our Health
by James Anderson
Per- and polyfluoroalkyl substances (PFAS) are a group of man-made chemicals that have been used in various industrial applications and consumer products since the 1950s. Known for their water, grease, and stain-resistant properties, PFAS are found in items such as non-stick cookware, water-repellent clothing, firefighting foams, and many other products. However, the widespread use and persistence of these chemicals in the environment have raised significant health and environmental concerns. This article explores the types of PFAS, their impacts on the environment and human health, current regulations, preventive measures, and potential alternatives.
PFAS encompass a large family of chemicals, with over 4,700 identified compounds. The most well-known and studied PFAS are perfluorooctanoic acid (PFOA) and perfluorooctanesulfonic acid (PFOS), which have been widely used in various applications. Other notable PFAS include GenX chemicals, perfluorobutane sulfonate (PFBS), and perfluorohexane sulfonate (PFHxS).
Perfluorooctanoic Acid (PFOA): PFOA, also known as C8, is a synthetic chemical used in the production of Teflon and other non-stick coatings. It is highly persistent in the environment and has been linked to numerous health issues.
Perfluorooctanesulfonic Acid (PFOS): PFOS has been used in various products, including firefighting foams, stain repellents, and water-resistant fabrics. Similar to PFOA, PFOS is highly persistent and bioaccumulative, leading to significant environmental and health concerns.
GenX Chemicals: GenX chemicals are a newer class of PFAS developed to replace PFOA. However, studies have shown that they may pose similar health risks and environmental persistence issues.
Other PFAS:Other PFAS, such as PFBS and PFHxS, are used in various industrial applications and consumer products. While they are considered to have shorter environmental half-lives compared to PFOA and PFOS, they still pose significant concerns due to their persistence and potential health effects.
Environmental Impact of PFAS
Persistence and Bioaccumulation: PFAS are often referred to as “forever chemicals” because of their extreme persistence in the environment. They do not break down naturally and can accumulate in soil, water, and living organisms. This persistence leads to widespread contamination, as PFAS can travel long distances through water and air, impacting remote and urban areas alike (Cousins et al., 2016).
Water Contamination: One of the most significant environmental concerns related to PFAS is water contamination. PFAS have been detected in drinking water sources around the world, posing a risk to both human health and aquatic ecosystems. These chemicals can enter water bodies through industrial discharges, landfill leachates, and the use of firefighting foams (Sun et al., 2016).
Soil Contamination: PFAS contamination in soil can occur through the application of contaminated biosolids as fertilizer, atmospheric deposition, and spills of PFAS-containing products. Contaminated soil can act as a long-term source of PFAS to groundwater and surface water, exacerbating the contamination of these resources (Ghisi et al., 2019).
Impact on Wildlife: PFAS have been found to bioaccumulate in various wildlife species, including fish, birds, and mammals. High levels of PFAS in wildlife can lead to reproductive, developmental, and immune system impairments, ultimately affecting population health and biodiversity (Giesy & Kannan, 2001).
Worse Environmental Impacts
Ecosystem Disruption: The persistence of PFAS in the environment can lead to the disruption of entire ecosystems. Bioaccumulation and biomagnification through the food chain mean that top predators, including birds of prey, marine mammals, and human beings, are at risk of high exposure levels. This not only threatens biodiversity but also the stability of ecosystems reliant on a balance of species (Rumsby et al., 2009).
Soil Fertility: PFAS contamination can significantly impact soil fertility, affecting agricultural productivity. Contaminated soils may inhibit the growth of crops and plants, leading to reduced yields and economic losses for farmers. The chemicals can also enter the human food chain through contaminated crops, posing further health risks (Ghisi et al., 2019).
Health Impact of PFAS
Human Exposure: Humans can be exposed to PFAS through multiple pathways, including ingestion of contaminated food and water, inhalation of dust, and dermal contact with PFAS-containing products. Due to their widespread use and persistence, virtually everyone has some level of PFAS in their blood (Agency for Toxic Substances and Disease Registry, 2020).
Health Effects: PFAS exposure has been linked to various adverse health effects, including:
– Cancer: Studies have shown associations between PFAS exposure and increased risks of kidney and testicular cancer (Barry et al., 2013).
– Hormonal Disruption: PFAS can interfere with thyroid function and hormone levels, leading to developmental and reproductive issues (Jain, 2013).
– Immune System Effects: PFAS exposure has been linked to reduced vaccine efficacy and increased susceptibility to infections (Grandjean et al., 2012).
– Liver Damage: PFAS can cause liver toxicity and increase the risk of liver disease (Borg et al., 2013).
– Cholesterol Levels: Elevated levels of PFAS are associated with higher cholesterol levels, which can contribute to cardiovascular diseases (Nelson et al., 2010).
Worse Health Impacts
Developmental Issues: PFAS exposure during pregnancy can lead to developmental issues in infants, including low birth weight, early puberty, and skeletal variations. These developmental impacts can have long-term consequences for children’s health and development, affecting their physical and cognitive abilities (Fei et al., 2007).
Metabolic Disorders: There is growing evidence that PFAS exposure may contribute to metabolic disorders such as obesity and diabetes. PFAS can interfere with lipid metabolism and glucose homeostasis, potentially leading to increased risk of metabolic syndrome and related conditions (Lind et al., 2014).
Neurotoxicity: Recent studies suggest that PFAS may have neurotoxic effects, impacting brain development and function. This can lead to behavioral and cognitive deficits, increasing the risk of neurodevelopmental disorders such as ADHD and autism spectrum disorder (Oulhote et al., 2016).
Regulations and Preventive Measures
International Regulations: International efforts to regulate and manage PFAS are ongoing, with various countries implementing measures to limit their production and use.
Stockholm Convention: The Stockholm Convention on Persistent Organic Pollutants (POPs) has listed PFOS and PFOA for global restriction and elimination. Countries that are parties to the convention are required to take measures to eliminate or restrict the production and use of these chemicals (Stockholm Convention, 2019).
National Regulations: Different countries have established regulations to control PFAS contamination and protect public health.
United States: In the United States, the Environmental Protection Agency (EPA) has issued health advisories for PFOA and PFOS in drinking water and is working on establishing enforceable limits. The EPA’s PFAS Action Plan outlines steps to address PFAS contamination, including monitoring, research, and regulatory actions (EPA, 2019).
European Union: The European Union has taken significant steps to regulate PFAS, including restrictions on the use of PFOS and PFOA under the Registration, Evaluation, Authorisation and Restriction of Chemicals (REACH) regulation. Additionally, the EU is considering further restrictions on a broader group of PFAS (European Chemicals Agency, 2017).
Preventive Measures
Source Control: Preventing PFAS contamination at the source is the most effective way to protect human health and the environment. This includes phasing out the use of PFAS in consumer products, industrial processes, and firefighting foams. Many companies have already begun transitioning to safer alternatives (Cousins et al., 2019).
Water Treatment: Advanced water treatment technologies, such as granular activated carbon (GAC), ion exchange resins, and high-pressure membranes (e.g., reverse osmosis), can effectively remove PFAS from drinking water. These technologies are essential for treating contaminated water sources and ensuring safe drinking water supplies (Rahman et al., 2014).
Soil Remediation: Soil remediation techniques, such as excavation and disposal, soil washing, and in situ stabilization, can help address PFAS-contaminated sites. Emerging technologies, such as thermal desorption and bioremediation, show promise for effectively treating PFAS-contaminated soils (Ross et al., 2018).
Public Awareness: Raising public awareness about PFAS and their potential health risks is crucial for promoting safer consumer choices and supporting regulatory actions. Public education campaigns and transparent communication about PFAS contamination and mitigation efforts can empower communities to take action and demand safer alternatives.
Alternatives to PFAS
Non-Fluorinated Alternatives: Developing and adopting non-fluorinated alternatives to PFAS is critical for reducing environmental and health risks. Non-fluorinated alternatives include:
– Silicone-Based Compounds: These compounds offer water and oil repellency without the persistence issues associated with PFAS. They are used in applications such as textiles and food packaging (Llorca et al., 2013).
– Waxes and Bio-Waxes: Natural and synthetic waxes can provide water repellency for paper and textile products. Bio-waxes derived from renewable sources are particularly attractive for their sustainability (Hansen et al., 2010).
– Zinc Oxide Nanoparticles: These nanoparticles can be used as an alternative to PFAS in some applications, offering similar water and oil repellency properties (Guzman-Puyol et al., 2017).
Green Chemistry: Green chemistry principles aim to design chemical products and processes that reduce or eliminate the use and generation of hazardous substances. Applying these principles to develop PFAS alternatives can lead to safer and more sustainable products (Anastas & Warner, 1998).
Research and Development: Ongoing research and development efforts are crucial for identifying and testing new PFAS alternatives. Collaboration between industry, academia, and regulatory agencies can accelerate the development of safe and effective substitutes for PFAS (Grandjean & Clapp, 2015).
Case Studies
Case Study 1: GenX Contamination in North Carolina
In North Carolina, the Chemours Company’s Fayetteville Works facility has been identified as a source of GenX contamination in the Cape Fear River. GenX chemicals, used as a replacement for PFOA, were found in the drinking water of over 250,000 residents. The contamination has raised concerns about the safety and environmental impact of GenX chemicals, leading to regulatory actions and public outcry (Sun et al., 2016).
Case Study 2: PFAS in Firefighting Foams
Aqueous film-forming foams (AFFFs) containing PFAS have been widely used for firefighting, particularly at military bases and airports. These foams have led to significant PFAS contamination in groundwater and drinking water sources. In response, many countries are phasing out PFAS-based foams and adopting fluorine-free alternatives to mitigate environmental and health risks (Schultz et al., 2004).
Case Study 3: The PFAS-Free Campaign in the UK
The PFAS-Free campaign in the UK aims to raise awareness about the risks associated with PFAS and advocate for the use of safer alternatives. The campaign has successfully influenced policy changes and encouraged companies to transition to non-fluorinated alternatives. This grassroots initiative demonstrates the power of public advocacy in driving regulatory and industry actions (PFAS-Free, 2021).
PFAS pose significant challenges due to their persistence, bioaccumulation, and potential health risks. Addressing PFAS contamination requires a comprehensive approach that includes stringent regulations, preventive measures, and the development of safer alternatives. By raising public awareness, promoting green chemistry, and investing in research and development, we can reduce our reliance on PFAS and protect both human health and the environment.
References
- Adams, K. T., Osmani, M., Thorpe, T., & Thornback, J. (2017). Circular economy in construction: Current awareness, challenges, and enablers. Proceedings of the Institution of Civil Engineers – Waste and Resource Management, 170(1), 15-24.
- Agency for Toxic Substances and Disease Registry. (2020). Per- and Polyfluoroalkyl Substances (PFAS) and Your Health. Retrieved from [ATSDR](https://www.atsdr.cdc.gov/pfas/index.html)
- Anastas, P. T., & Warner, J. C. (1998). Green Chemistry: Theory and Practice. Oxford University Press.
- Barry, V., Winquist, A., & Steenland, K. (2013). Perfluorooctanoic acid (PFOA) exposures and incident cancers among adults living near a chemical plant. Environmental Health Perspectives, 121(11-12), 1313-1318.
- BASF. (2020). ChemCycling: Innovative chemical recycling of plastics. Retrieved from [BASF](https://www.basf.com/global/en/who-we-are/sustainability/we-drive-sustainable-solutions/circular-economy/chemcycling.html)
- Borg, D., Lund, B. O., Lindquist, N. G., & Håkansson, H. (2013). Cumulative health risk assessment of 17 PFASs in the Swedish population. Environmental International, 59, 112-123.
- Braskem. (2019). Green Polyethylene: Renewable plastics from sugarcane. Retrieved from [Braskem](https://www.braskem.com.br/portal/green-polyethylene)
- Cousins, I. T., Goldenman, G., Herzke, D., Lohmann, R., Miller, M., Ng, C. A., … & Wang, Z. (2019). The concept of essential use for determining when uses of PFASs can be phased out. Environmental Science: Processes & Impacts, 21(11), 1803-1815.
- Cousins, I. T., Vestergren, R., Wang, Z., Scheringer, M., & McLachlan, M. S. (2016). The precautionary principle and chemicals management: The example of perfluoroalkyl acids in groundwater. Environment International, 94, 331-340.
- European Chemicals Agency. (2017). Annex XV Restriction Report: Proposal for a Restriction on Perfluorooctanoic Acid (PFOA), its Salts and PFOA-Related Substances. Retrieved from [ECHA](https://echa.europa.eu/documents/10162/4d3da5da-e67f-4d7a-b0ae-5e7abac90fc0)
- Environmental Protection Agency. (2019). EPA’s Per- and Polyfluoroalkyl Substances (PFAS) Action Plan. Retrieved from [EPA](https://www.epa.gov/pfas/epas-pfas-action-plan)
- Fei, C., McLaughlin, J. K., Tarone, R. E., & Olsen, J. (2007). Perfluorinated chemicals and fetal growth: A study within the Danish National Birth Cohort. Environmental Health Perspectives, 115(11), 1677-1682.
- Giesy, J. P., & Kannan, K. (2001). Global distribution of perfluorooctane sulfonate in wildlife. Environmental Science & Technology, 35(7), 1339-1342.
- Ghisi, R., Vamerali, T., & Manzetti, S. (2019). Accumulation of perfluorinated alkyl substances (PFAS) in agricultural plants: A review. Environmental Research, 169, 326-341.
- Grandjean, P., Andersen, E. W., Budtz-Jørgensen, E., Nielsen, F., Mølbak, K., Weihe, P., & Heilmann, C. (2012). Serum vaccine antibody concentrations in children exposed to perfluorinated compounds. JAMA, 307(4), 391-397.
- Grandjean, P., & Clapp, R. (2015). Changing interpretation of human health risks from perfluorinated compounds. Public Health Reports, 130(4), 321-323.
- Guzman-Puyol, S., Heredia-Guerrero, J. A., Ceseracciu, L., Dante, S., Bayer, I. S., Heredia, A., & Athanassiou, A. (2017). Bioinspired transition-metal-doped nanoparticle-based coatings for durable hydrophobic and antibacterial properties. Scientific Reports, 7(1), 16980.
- Hansen, K. J., Clemen, L. A., Ellefson, M. E., & Johnson, H. O. (2010). Compound-specific, quantitative characterization of organic fluorochemicals in biological matrices. Environmental Science & Technology, 35(4), 766-770.
- Jain, R. B. (2013). Association between thyroid function and selected perfluoroalkyl acids in adolescents aged 12-19 years: NHANES 2007-2010. Environmental Research, 126, 51-59.
- Lind, L., Zethelius, B., Salihovic, S., van Bavel, B., & Lind, P. M. (2014). Circulating levels of perfluoroalkyl substances and prevalent diabetes in the elderly. Diabetologia, 57(3), 473-479.
- Llorca, M., Pérez, F., Farré, M., & Barceló, D. (2013). Analysis of perfluoroalkyl substances in Spanish sewage sludge by pressurized solvent extraction followed by liquid chromatography–mass spectrometry. Journal of Chromatography A, 1290, 45-53.
- Nelson, J. W., Hatch, E. E., & Webster, T. F. (2010). Exposure to polyfluoroalkyl chemicals and cholesterol, body weight, and insulin resistance in the general U.S. population. Environmental Health Perspectives, 118(2), 197-202.
- Oulhote, Y., Pizot, C., & Weisskopf, M. G. (2016). Perfluoroalkyl chemicals and child and adolescent behavior. Environmental Research, 147, 133-144.
- PFAS-Free. (2021). About PFAS. Retrieved from [PFAS-Free](https://www.pfasfree.org.uk/about-pfas)
- Rahman, M. F., Peldszus, S., & Anderson, W. B. (2014). Behaviour and fate of perfluoroalkyl and polyfluoroalkyl substances (PFASs) in drinking water treatment: A review. Water Research, 50, 318-340.
- Ross, I., Hurst, J., Miles, J., & Kalve, E. (2018). PFAS in Soil and Groundwater: Remediation Technologies. In Perfluoroalkyl Substances in the Environment (pp. 37-61). Springer.
- Rumsby, P. C., Hall, T., & Infante, H. G. (2009). Perfluorooctane sulphonate and perfluorooctanoic acid in drinking and environmental waters. Philosophical Transactions of the Royal Society A: Mathematical, Physical and Engineering Sciences, 367(1904), 4119-4136.
- Schultz, M. M., Barofsky, D. F., & Field, J. A. (2004). Quantitative determination of fluorotelomer sulfonates in groundwater by LC MS/MS. Environmental Science & Technology, 38(6), 1828-1835.
- Stockholm Convention. (2019). The New POPs under the Stockholm Convention. Retrieved from [Stockholm Convention](http://chm.pops.int/Implementation/NewPOPs/Overview/tabid/6678/Default.aspx)
- Sun, M., Arevalo, E., Strynar, M. J., Lindstrom, A. B., Richardson, M., Kearns, B., … & Knappe, D. R. (2016). Legacy and emerging perfluoroalkyl substances are important drinking water contaminants in the Cape Fear River watershed of North Carolina. Environmental Science & Technology Letters, 3(12), 415-419.